WP3
Lead – Ryan Hossaini, University of Lancaster
Because of their rapid growth rate and potential to substantially delay ozone layer recovery, it is vital to understand the emissions and atmospheric impacts of unregulated halocarbons with relatively short lifetimes (< 6 months). InHALE will quantify emissions from industrial and oceanic sources of these substances and better quantify the impacts of these VSLS on the ozone layer.
WP 3.1: Ocean production and emission of dichloromethane and chloroform (York)
Ocean emissions may account for ~50% of total chloroform emissions (Worton et al., 2006) and are a highly uncertain term in the global budget of both chloroform and dichloromethane (e.g., Moore 2004). Even relatively small oceanic emissions have the potential to contribute to the Upper Troposphere Lower Stratosphere (UTLS) chlorine budget if occurring in the tropics. InHALE will collect new air and seawater Cl-VSLS measurements at Cape Verde to investigate in situ ocean production and to constrain NH tropical ocean emissions.
In collaboration with the Cape Verde Instituto do Mar (Imar) and the GEOMAR-Helmholtz Centre for Ocean Research, Kiel (see LoS), we will collect monthly seawater samples at the Cape Verde Ocean Observatory (CVOO, ~100 km northeast of the CVAO) and along a transect to the CVOO via the routine ship sampling organised by GEOMAR. Discrete surface seawater will be sampled from Niskin bottles attached to a CTD-rosette. Samples will be analysed in duplicate within 24 hours (stored at seawater temperature) by purge and trap GC-MS in the Imar laboratory by a CVOO technician trained and equipped by York. We envisage 5 seawater samples per trip at different (but fixed) locations between the CVAO and CVOO to ensure sampling across a range of biological activity. Sampling will continue for 18-24 months. Analysis of CFC-11 concentrations alongside those of dichloromethane and chloroform will allow calculation of the excess dichloromethane and chloroform saturation anomalies, required to assess whether in-situ production is occurring (Ooki and Yokouchi, 2011). Natural VSLS (CH3I, CHBr3, etc.) will also be analysed. Saturation anomalies will be analysed for patterns and trends that correlate with, for example, chlorophyl-A, coloured dissolved organic material and dissolved organic carbon abundances, as determined from satellite products or from CVOO sampling, and natural VSLS. To determine sea-air fluxes, we will use air samples from the cruises or air measurements from CVAO. If in-situ production is occurring, we will explore a parameterisation with co-measured variables to produce new tropical – subtropical ocean flux estimates.
WP 3.2: Global and regional VSLS emissions (Lancaster, Cambridge, Bristol)
Prominent industrial Cl-VSLS include dichloromethane, a solvent used in a range of emissive applications and as a feedstock to produce HFC-32, and chloroform, a feedstock in HCFC-22 production. In the NH, surface dichloromethane has increased by a factor of ~2.5 since the early 2000s (Figure 7). Emissions from China account for ~50-60% of the global total (An et al., 2021), and aircraft campaigns suggest that Indian emissions increased 5-fold between 2008 and 2016 (Leedham Elvidge et al., 2015; Say et al., 2019), but this has not been confirmed using long-term surface measurements. Emissions of chloroform have also risen dramatically over the last decade, due, primarily, to growing emissions from eastern Asia (Fang et al., 2018).
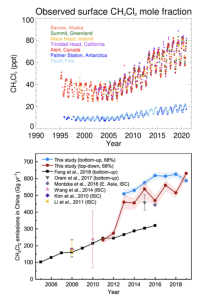
We will develop gridded datasets describing surface emissions of key industrial Cl-VSLS (dichloromethane, chloroform, C2Cl4), and 1,2- dichloroethane, newly measured through WP 1.2. Emissions will be inferred using both bottom- up and top-down approaches.
Bottom-up estimates, provided by consultant Sherry, will utilise detailed country-level data on annual Cl-VSLS production from major emitting regions, and species-specific data on emissive versus feedstock uses.
To compare to these bottom-up estimates, two sets of inversions will be performed: global ‘synthesis inversions’ using TOMCAT for the period mid-1990s to 2025 (e.g., Claxton et al., 2020), and regional HBMCMC inversions using NAME HBMCMC and the time-resolved footprints developed in WP 2.3 for the period 2010 to 2025 (e.g., An et al., 2021). The inversions will benefit from assimilating the new harmonised NOAA and AGAGE global network data (see WP 1.3), along with new data from the CVAO site (important given its tropical location) and new InHALE measurements in East and South Asia. The inversions will incorporate into their a priori constraints the new bottom-up anthropogenic fluxes developed in this WP, and ocean fluxes from WP 3.1. The estimates from the different inversions will be intercompared in key regions such as South and East Asia to better understand uncertainties, and global emission estimates will be used to understand limitations of the global estimates provided by the AGAGE 12-box model (widely used in WMO Ozone Assessments and elsewhere).
WP 3.3: Delivery of Cl-VSLS and their products to the stratosphere (Lancaster, Leeds, Cambridge)
Global models indicate Cl-VSLS growth may have slowed the rate at which upper stratospheric HCl is declining by ~15%, offsetting some of the MP’s benefits (Hossaini et al., 2019). Tropospheric transport processes and chemical sinks strongly influence how efficiently VSLS emissions reach the stratosphere, and this is an area with significant uncertainty (Hossaini et al., 2016).
InHALE will use four state-of-the-art global models to perform the first concerted assessment of historical Cl-VSLS impacts from the surface to the stratosphere, including an up-to-date assessment of impacts on polar and extra-polar ozone and ozone trends. Optimised global emissions from WP 3.1 and WP 3.2 will be implemented in TOMCAT and UKCA, along with our partner models WACCM (Kinnison LoS), CAM-Chem (Saiz-Lopez LoS) and EMAC (Sinnhuber LoS). We will evaluate the ability of the models to capture Cl-VSLS observations in the UTLS using data from historical NASA missions (e.g., ATTREX, POSIDON) and recent data in the important Asian Summer Monsoon region (Adcock et al., 2021). A common set of transient simulations covering the mid-1990s to present will be performed, providing a multi-model assessment of the time-varying stratospheric impacts of Cl-VSLS growth. We will: (1) quantify the source gas versus product gas injection of chlorine to the stratosphere from C-VSLS, (2) test the hypothesis that Cl-VSLS have slowed the rate at which total stratospheric chlorine is declining (Hossaini et al., 2019), utilising vertically-resolved satellite HCl observations (e.g., public ACE-FTS data), and (3) determine the contribution of Cl-VSLS to polar and extra-polar ozone depletion, including their effect in recent remarkable cold Arctic winters. A comprehensive sensitivity analysis will be carried out by TOMCAT and UCKA to assess how our findings vary with, for example, model horizontal and vertical resolution, and the choice of chemical mechanism (and hence tropospheric hydroxyl radical concentrations).
WP 3.4: Metrics to compare ozone depletion (Lancaster, Leeds, Cambridge)
Ozone depletion potential (ODP) is an important metric, widely adopted to assess the ozone impact of an industrial release of a gas compared to a reference. Traditionally, the ODP of a gas is defined as the ratio of the steady state total column ozone decrease due to an emission, over the total column ozone decrease due to the same mass emission of CFC-11. Although a cornerstone to the MP, few ODP estimates for industrial Cl-VSLS exist. Additionally, because Cl-VSLS break down and affect ozone in the troposphere, a revised stratospheric ODP (SODP) has been suggested, considering ozone changes above the tropopause only (Zhang et al., 2020). InHALE will quantify the ODP and SODP metrics for a range of industrial VSLS, evaluated using a range of models.
Time-slice experiments with TOMCAT, UCKA, WACCM, CAM-Chem and EMAC will quantify the steady state ODP and SODP of a wide range of industrial VSLS, including emerging compounds (e.g.,1,2-dichloropropane, 1,2-dichlorobenzene) reported to be elevated in Asia (Lyu et al., 2020). We will first quantify both metrics for present day conditions, testing their sensitivity to a range of emission patterns (e.g., including a scenario with surface emissions dominated by east Asia). As interannual variability affects VSLS troposphere-to-stratosphere transport, both metrics will be evaluated under a range of meteorological conditions. We will test the sensitivity of SODP to assumptions around tropopause definition. Due to changes to the stratosphere’s background composition, ODPs/SODPs are unlikely static in time (Klobas et al., 2020), thus both metrics will also be quantified under 2050 conditions, defined by a range of Shared Socioeconomic Pathways (SSP).
WP 3.5: Future scenarios (Sherry, Lancaster)
Unlike controlled ODSs, there has been no attempt to project industrial Cl-VSLS emissions which are essential for credible estimates of the delay to ozone recovery. Informed by in-depth industry- specific information, InHALE will develop projections of the possible future evolution of key Cl-VSLS emissions from major industrialised regions.
With Sherry (consultant), we will synthesise factors thought to affect future Cl-VSLS markets and create narrative-based quantitative scenarios describing future industrial dichloromethane, chloroform, and 1,2-dichloroethane emissions. Projections to the year 2050 will be based on anticipated changes in the production of regulated ODSs (e.g., HCFC-22), demand for Cl-VSLS as feedstocks (e.g., chloroform for PTFE, 1,2-dichloroethane for PVC) and solvents, and evolving industry practice. In addition to a business-as-usual case, we will explore futures with relatively low and high Cl-VSLS, the former characterised by e.g., increased toxicological awareness around Cl- VSLS (e.g., dichloromethane) in Asia and/or increased solvent vapour recovery, the latter by stronger growth in the markets of PTFE and PVC plastics and increased industrialisation of wider SE Asia. The scenarios will be used to explore the impact of Cl-VSLS on ozone layer recovery, among other factors, in WP 5.
References
Adcock et al., 2021, J. Geophys. Res. Atmos., 126, e2020JD033137;
An et al., 2021. Nat. Commun., 12, 7279;
Claxton et al., 2020. J. Geophys. Res. Atmos., 125, e2019JD031818
Fang et al., 2019. Nature Geosci., 12, 89-93
Hossaini et al., 2016. Atmos. Chem. Phys., 16, 9163-9187
Hossaini et al., 2019. J. Geophys. Res. Atmos., 124, 2318-2335.
Klobas et al., 2020, Atmos. Chem. Phys., 20, 9459-9471;
Leedham Elvidge et al., 2015, Atmos. Chem. Phys., 15, 1939-1958;
Lyu et al., 2020. Chemosphere, 246, 125731;
Moore, 2004. J. Geophys. Res. Oceans., 109, C09004
Ooki and Yokouchi, 2011, Mar. Chem., 124, 119-124
Worton et al., 2006. Atmos. Chem. Phys., 6, 2847-2863
Say et al., 2019, Atmos. Chem. Phys., 19, 9865-9885;
Zhang et al., 2020. J. Geophys. Res. Atmos., 125, e2020JD032414.