WP5
Lead – Keith Shine, University of Reading
To accurately determine the full impacts of the developments described in WPs 1 – 4, new research is needed to quantify the influence of different halocarbons on ozone depletion and climate. This work package focuses on radiative forcing and ozone depletion impacts and the associated metrics required to put halocarbons in the context of other GHGs and ODSs.
WP 5.1: Halocarbon radiative efficiencies and forcings (Reading, RAL)
Calculation of the GWP for each halocarbon requires knowledge of the gas lifetime and its radiative efficiency (RE), which is the RF per unit concentration change. The RE relies on laboratory- measured (and sometimes theoretically calculated) infrared absorption cross-sections and detailed radiative transfer calculations, as well as knowledge of each halocarbon’s vertical and horizontal distribution. To date, values of RE for many halocarbons (including in Hodnebrog et al. (2020a) which is used in IPCC AR6) have mostly employed simplified vertical distributions, based on gas lifetime. The increasing availability of satellite observations (e.g., Hegglin et al., 2021) and of vertically and seasonally resolved model distributions, makes a more detailed analysis of RF and RE using these distributions timely. Some spectroscopic data for RE calculations are readily available (Gordon et al., 2022), but not yet used for this purpose and InHALE will also fill gaps in spectroscopic data by making its own targeted laboratory measurements. InHALE will also assess RF impact of HFO degradation products from WP 4, and of ODS degradation products.
Narrow-band (Shine and Myhre, 2020) and line-by-line (Dudhia, 2017) radiative transfer codes will be used to: (i) exploit recent satellite data (MIPAS and ACE-FTS from the SPARC Data Initiative, Hegglin et al. 2021) to quantify the impact of using more refined halocarbon distributions on RE and compare with simplified approaches used in recent IPCC assessments, (ii) compute REs of halocarbon degradation products, including HCl, ClONO2 and CF2O, exploiting satellite data and the advances from WP 4, (iii) include solar near-infrared absorption in RE calculations, which has been neglected to date but may modify the effect of lighter halocarbons. Parallel calculations for some species will performed by project partner CICERO to enable intercomparisons.
We will mostly utilise extensive spectroscopic data catalogued on the HITRAN database (Gordon et al. 2022) but not all gases of interest in InHALE have adequate measurements, because of insufficient wavelength coverage, pressure and temperature conditions that are inadequate for atmospheric purposes, disagreements in the literature, or the emergence of newly identified species for which data is not yet available. InHALE will use the RAL High-Resolution Spectroscopy Facility to acquire and analyse new measurements and incorporate these in the radiative transfer codes and make the measurements available for future HITRAN versions.
We will test the extent to which lower-resolution radiative transfer codes used in ESMs reproduce the forcing and heating rate perturbations that result from changing halocarbon concentrations in 15 more detailed models. These perturbations are important in driving the rapid adjustments in ESMs (below).
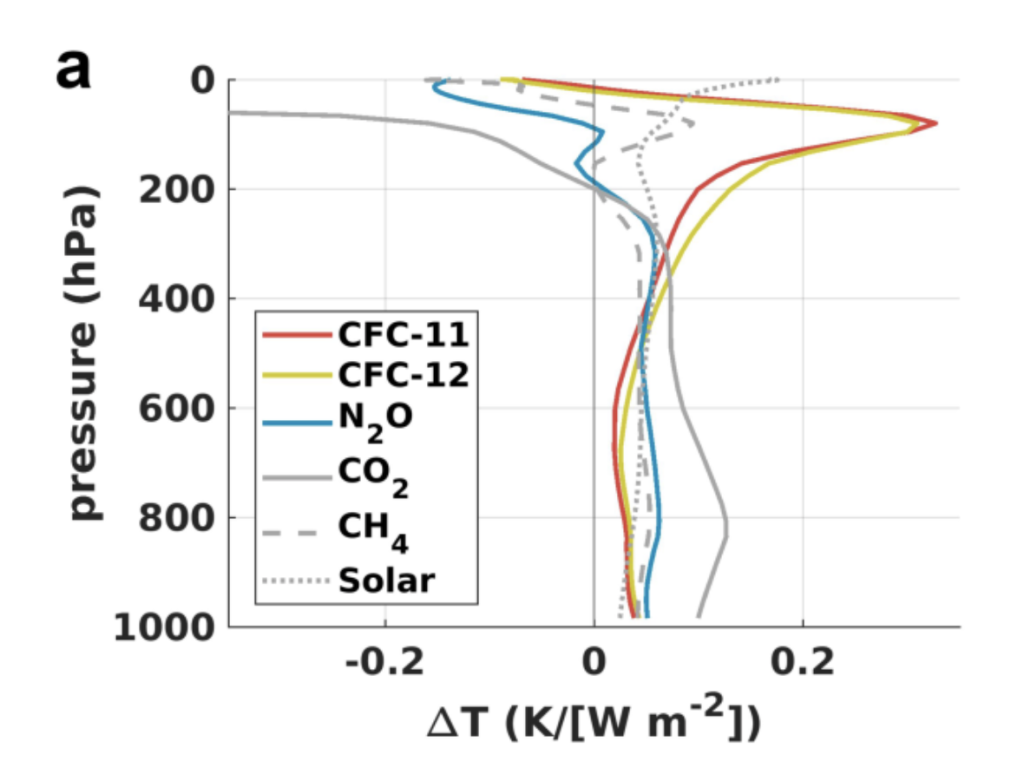
WP 5.2: Effective radiative forcing and rapid adjustments (Reading, Cambridge)
The preferred measure of RF in IPCC AR6 is now Effective RF (ERF) that accounts for rapid atmospheric adjustments in the absence of surface temperature change. This includes, for example, changes to tropospheric (as well as stratospheric) temperature, cloud properties and stratospheric water vapour. Calculation of ERF requires the use of Earth System Models (ESMs), which have less detailed radiative transfer codes than normally used for RF calculations and substantial internal variability, which makes small perturbations difficult to detect. Hodnebrog et al. (2020b), which considered CFC-11 and CFC-12, was the only study that IPCC AR6 (Forster et al., 2021) drew on for in its “low confidence” enhancement of halocarbon ERF values by 12%. This showed clearly that halocarbon RAs are very distinct to those from other greenhouse gases, most notably in the UTLS (see image above). Through a set of ESM runs by InHALE and partners, we will build a more comprehensive understanding of halocarbon rapid adjustments, and assess the extent to which results for, say, CFC-11, can be applied to other halocarbons.
Because quantification of rapid adjustments (RAs) using ESMs for halocarbons is in its infancy, we will examine the extent to which there are generic RAs common to all halocarbons. A particular focus will be the RAs caused by halocarbon-induced changes in ozone and stratospheric water vapour, both of which will differ significantly between halocarbons. Because of the computational expense of ESM integrations, the aim is to build process-level understanding that can be applied more generally in WP 5.4. The primary tool will be the joint Met Office – NERC UKESM1, incorporating UK Chemistry and Aerosol (UKCA-CRIStrat) chemistry (Weber et al., 2021). For a subset of proposed experiments, project partners (CICERO and NCAR – see LoS from Måseide and Kinnison) will perform parallel experiments with their ESMs to enable intercomparisons. Diagnostics will include double-call radiation calculations which will help isolate the role of particular constituents in the ERF and radiative kernels, which can isolate the role of, for example, cloud adjustments.
The species analysed will be CFC-11, CFC-12, HCFC-22, HCFC-141b, HFC-134a and a VSLS (dichloromethane or chloroform), contingent on results of their impact on ozone loss from WP 3. The chosen species have a range of tropospheric and stratospheric lifetimes and impacts on stratospheric ozone. The direct climate impact will use perturbations to selected species sufficient to give an ERF of order 1 W m-2 (with all non-halogen GHGs at 2020 concentrations) with the radiation scheme only responding to the changes in the perturbed species. For ODSs, the climate impact of the ozone depletion will be isolated using 2020 concentrations of the selected species, but with the model radiation scheme only responding to ozone changes.
Experiments will be 40-year integrations with fixed sea-surface temperatures. Species perturbations will be incorporated via a fixed surface boundary condition, so that the vertical and horizontal distribution is determined by the model. Further experiments (about 6) will test additivity and linearity of RAs. These experiments will be chosen based on results of the single species experiments above, but could include larger CFC-11 perturbations, combined CFC-11+HCFC-22 and combined CFC- 11+HCFC-22+HFC-134a experiments.
WP 5.3: Halocarbon climate impacts (Reading, Cambridge)
ESMs are needed to assess halocarbon-driven climate change beyond ERF and surface temperature. Again, there is a paucity of studies, but those that are available indicate that halocarbon impacts can be disproportionately large, relative to what would be anticipated from their ERF alone (e.g., substantial upper tropospheric warming (McLandress et al., 2014), Arctic amplification (Liang et al., 2022) or an acceleration of the Brewer-Dobson circulation (Hegglin and Shepherd, 2009)). We will carry out 150-year step-change ESM experiments, with interactive oceans and chemistry, performed with perturbations of a single halocarbon species, imposed via surface boundary conditions. Two species (CFC-11 and HCFC-22) provide a range of lifetimes and ODPs and are expected to lead to distinct climate responses which will help InHALE to build generic understanding. As in WP 5.2, separate experiments will be performed in which the radiation code just sees the direct response or just sees the ozone response. Results from WP 5.2 will be used to select perturbations which will generate detectable signals. These integrations will be analysed in conjunction with WP 5.2, to distinguish changes due to rapid adjustments and those mediated by surface temperature change. Diagnosis will focus on surface, troposphere, and stratospheric temperature changes, including Arctic amplification, dynamical indicators such as tropopause height and temperature, the strength of the Brewer-Dobson circulation, tropical broadening, changes in upper-tropospheric jet structure and associated modes and hydrological cycle response.
WP 5.4: Improved GWPs and other emission metrics (Reading, Cambridge)
This WP will integrate outputs from throughout InHALE including WPs 4.2, 5.1 and 5.2 to calculate improved GWPs and selected other metrics such as the Global Temperature-change Potential, catalogued in IPCC AR6 (Forster et al. 2021). It will use refined values of effective RE and go well beyond previous (e.g., IPCC) tabulations by including explicit consideration of halocarbon degradation products, in addition to indirect forcings computed in WP 5.2.
Radiative efficiencies will be incorporated into a simple climate model (e.g., the FAIR model of Smith et al. 2018) to provide an updated assessment of the past and possible future impacts of halocarbon emissions on surface temperature, integrating the improved understanding of these emissions from InHALE (WP 2 and WP 3).
WP 5.5: Ozone-climate interactions and implications for ozone recovery (Leeds, Cambridge, Lancaster)
Even though the first signs of ozone layer recovery have been detected (e.g., Chipperfield et al., 2017) there is still significant uncertainty in how it will progress in different atmospheric regions, due to the coupling with climate change (e.g., due to changes in stratospheric temperatures and thus the occurrence of polar stratospheric clouds or an increase in the speed of the Brewer-Dobson circulation). InHALE will identify new climate feedbacks due to halocarbons, with special focus on the tropopause region, by modelling a wider range of compounds, and more realistic distributions and RFs than previous studies. The impact of these so-far-ignored feedbacks on the projected recovery of the ozone layer needs to be quantified using targeted simulations and put into the context of the direct impact of halocarbon emissions.
We will quantify the impact on ozone recovery of (i) alternative halocarbon (ODS and VSLS) scenarios and (ii) the climate impact of ODSs. The coupled chemistry ESM simulations from WPs 5.2 and 5.3 will be further analysed to determine projected recovery of ozone. These will be complemented by transient UKESM runs with VSLS scenarios from WP 3.5. The impact of different climate forcings on ozone will be determined.
Recently, Pyle et al. (2022) argued for the adoption of Integrated Ozone Depletion (IOD) as a better metric for ozone recovery, compared to the widely used date of return to 1980 levels. This IOD metric takes account of the full perturbation to the ozone layer and is directly relevant to assessing impacts such as surface UV. IOD can be calculated from models, but Pyle et al. (2022) showed that, at least for long-lived ODSs, a simple empirical expression can also be derived. InHALE will further explore the use of this metric, develop an empirical version also for VSLS and promote its use to policy makers and the research community. Values of IOD will be derived for the different ODS and VSLS scenarios used in the model. These will be used to test the empirical version of IOD for long-lived species and to adapt it for use with VSLS.
References
Chipperfield et al., 2017, Nature, 549, 211-218;
Dudhia 2017, J. Quant. Spectrosc. Radiat. Transf., 186, 243-253;
Gordon et al., 2022, J. Quant. Spectrosc. Radiat. Transf., 277, 107949;
Hegglin et al., 2021, Earth Syst. Sci. Data, 13, 1855-1903;
Hegglin and Shepherd, 2009, Nat. Geo., 2, 687-691;
Hodnebrog et al., 2020a, Rev. Geophys., 58, e2019RG000691;
Hodnebrog et al., 2020b, Npj Clim. Atmos. Sci., 3(1), 43;
Liang et al., 2022, Environ. Res. Lett., 17 (2), 024010;
McLandress et al., 2014; Atmos. Chem. Phys., 14, 15471555;
Pyle et al., 2022, Nature (in press)
Shine and Myhre, 2020, J. Adv. Model Earth Syst., 12, e2019MS001951;
Smith et al., 2018, Geosci. Model Dev., 11, 2273-2297;